Our Three Key Developments
One
The coatings for stable photocatalysis
Two
Design principles for achieving unity quantum efficiency
Three
Pioneering study of catalysis and adsorption under non-equilibrium chemical transport flux
We have created a catalyst discovery platform that combines coating-modified photocatalysts with flow devices to efficiently produce H2, syngas, and H2O2 solutions at scale. The H2O2 technology has been deployed at 1 m², with others currently being scaled up.
These semiconductor PEC devices can produce not only fuels such as hydrogen but also fine chemicals, including benzaldehyde. Additionally, they can facilitate water oxidation to produce hydrogen peroxide, selective CH4 partial oxidation to utilize hydrogen peroxide, and N2 oxidation to produce fertilizer and drug precursors, among other applications.
This catalyst design platform should accelerate the development of developing light-driven chemical production, an alternative to thermal catalysis.
Photoelectrochemistry
Photoelectrochemical Interface: A Novel platform for light/chemical transduction
Photocatalytic Surface
Photocatalytic Surface Chemistry: Adsorption coupled with photo-induced charge transfer
Molecular Flux Catalysis
Molecular Flux Catalysis: Non-equilibrium CO2 photocatalysis in flowing seawater
Coating + X
Multi-Functional Coatings: Novel compositions, processing, and scale-up manufacturing
PEC Device Scale-Up
PEC Device Scale-Up: Design and manufacturing for scalable PEC Systems
AI Hardware
Energy-Efficient Analogue Computing: Photoelectrochemical Neural Network
Our Research Approach
Advancing Semiconductor Catalysis: Integrating Theory, Modeling, and Operando Characterizations for Efficient Light-to-Chemical Conversion
We take a multi-disciplinary approach, integrating electron-transfer theory, method developments, materials science, and applied mathematics to advance semiconductor catalysis science. Our research scope is to elucidate particles operation by connecting charge dynamics inside particles to charge-transfer kinetics at particle/liquid interfaces. We are interested in finding out the governing rules of running a light-driven artificial photo-synthetic system efficiently and cost-effectively.
Therefore, our research scope is to elucidate photocatalyst operation by connecting charge dynamics inside particles to charge-transfer kinetics at particle/liquid interfaces. To support the effort of quantifying photoelectrochemical (PEC) interfaces, we are building a multi-physics model to eventually simulate these processes.
Additionally, we employ in situ microscopic and spectroscopic techniques to interrogate materials/electrolyte interfaces at meso-to-nano-scale. We characterize various types of PEC interfaces, especially those stabilized with protective coatings, so that we can eventually connect charge-transfer rate processes with semiconductor device physics.
Archived Research
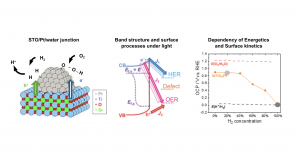
Particulate Photocatalysis
We, as a group, study the photocatalyst/(co-catalyst)/water interface and aim for rational design of efficient and stable photocatalytic systems.
Photocatalytic water-splitting involves light absorption, generation of electron–hole pairs, charge separation and bulk transport, charge transfer across interfaces for catalytic reactions, and mass transfer of reactants and products.
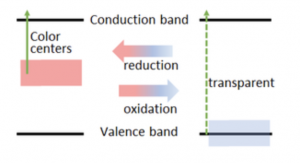
Multi-Functional Coating
We are developing multifunctional protective coatings, based on titanium dioxide (TiO2), for various photoelectrochemical synthesis pathways and electrochromic films. Different chemical reactions requires different thermodynamic driving forces, so it is important to tune your protective coatings to match your synthesis.
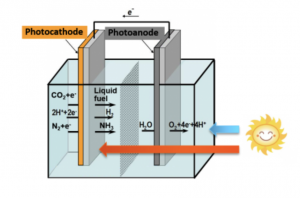
Stable Photoelectrochemical (PEC) Interface
Our strategy for durability is metal oxides based heterostructure interfaces in order to achieve efficient and stable photoelectrochemical water oxidation. We developed a new class of ternary oxide coating, (Ti,Mn)Ox, for efficient charge separation and stable water oxidation in acid.
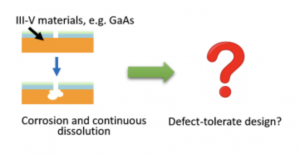
Operando Study
Through a systematic effort of monitoring photo-corrosion with operando microscopy and spectroscopy, we first understand typical photoanode corrosion process with a detailed description for the different corrosion stages. Secondly, we use our photocorrosion model to explain how various protection or corrosion behavior is manifested in shifts of surface corrosion potentials.
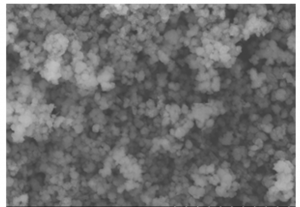
Tunable Nanomaterials for Catalysis
Our interests are also focusing on 2D tunable metal oxides. We collaborate with Prof. Lisa Pfefferle’s group. We use microscopy and spectroscopy to characterize materials/liquid interfaces under operating conditions.
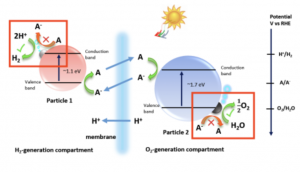
Modeling and Simulation
Chemical Engineers rely on modern modeling and simulation tools to design and optimize reactors. Solar-fuel reactors are a type of emerging chemical reactors.